Today we know that not only the first isotope of hydrogen (deuterium) produces fusion energy, but also the second (heavy) isotope of hydrogen (tritium) can produce energy by nuclear fusion.
Florian Ion T. Petrescu | Bucharest Polytechnic University
In nuclear fusion process two or more atomic nuclei join together, or "fuse", to form a single heavier nucleus. During this process, matter is not conserved because some of the mass of the fusing nuclei is converted to energy which is released. The binding energy of the resulting nucleus is greater than the binding energy of each of the nuclei that fused to produce it. This produces an enormous amount of energy.
Creating the required conditions for fusion on Earth is very difficult, to the point that it has not been accomplished at any scale for protium, the common light isotope of hydrogen that undergoes natural fusion in stars. In nuclear weapons, some of the energy released by an atomic bomb (fission bomb) is used for compressing and heating a fusion fuel containing heavier isotopes of hydrogen, and also sometimes lithium, to the point of "ignition". At this point, the energy released in the fusion reactions is enough to briefly maintain the reaction. Fusion-based nuclear power experiments attempt to create similar conditions using far lesser means, although to date these experiments have failed to maintain conditions needed for ignition long enough for fusion to be a viable commercial power source.
Research into developing controlled thermonuclear fusion for civil purposes also began in earnest in the 1950s, and it continues to this day. Two projects, the National Ignition Facility and ITER are in the process of reaching breakeven after 60 years of design improvements developed from previous experiments.
The best results were obtained with the Tokamak-type installations (see the Figures below).
ITER: the world's largest Tokamak
ITER is based on the 'tokamak' concept of magnetic confinement, in which the plasma is contained in a doughnut-shaped vacuum vessel. The fuel—a mixture of deuterium and tritium, two isotopes of hydrogen—is heated to temperatures in excess of 150 million°C, forming a hot plasma. Strong magnetic fields are used to keep the plasma away from the walls; these are produced by superconducting coils surrounding the vessel, and by an electrical current driven through the plasma.
The origin of the energy released in fusion of light elements is due to an interplay of two opposing forces, the nuclear force which draws together protons and neutrons, and the Coulomb force which causes protons to repel each other. The protons are positively charged and repel each other but they nonetheless stick together, portraying the existence of another force referred to as a nuclear attraction. Research into controlled fusion, with the aim of producing fusion power for the production of electricity, has been conducted for over 60 years. It has been accompanied by extreme scientific and technological difficulties, but has resulted in progress. At present, controlled fusion reactions have been unable to produce break-even (self-sustaining) controlled fusion reactions. Workable designs for a reactor that theoretically will deliver ten times more fusion energy than the amount needed to heat up plasma to required temperatures (see ITER) were originally scheduled to be operational in 2018, however this has been delayed and a new date has not been stated [2-7].
H-hour
In an anterior work [1] we spoke about the nuclear reactions.
The current nuclear power is considered a transition way, to the energy thermonuclear, based on fusion of light nuclei.
The main particularity of synthesis reaction (fusion) is the high prevalence of the used fuel (primary), deuterium. It can be obtained relatively simply from ordinary water.
Deuterium was extracted from water for the first time by Harold Urey in 1931. Even at that time, small linear electrostatic accelerators, have indicated that D-D reaction (fusion of two deuterium nuclei) is exothermic.
Today we know that not only the first isotope of hydrogen (deuterium) produces fusion energy, but also the second (heavy) isotope of hydrogen (tritium) can produce energy by nuclear fusion.
The first reaction is possible between two nuclei of deuterium, from which can be obtained, either a tritium nucleus plus a proton and energy, or an isotope of helium with a neutron and energy.
Observations: a deuterium nucleus has a proton and a neutron; a tritium nucleus has a proton and two neutrons.
Fusion can occur between a nucleus of deuterium and one of tritium.
Another fusion reaction can be produced between a nucleus of deuterium and an isotope of helium.
For these reactions to occur, should that the deuterium nuclei have enough kinetic energy to overcome the electrostatic forces of rejection due to the positive tasks of protons in the nuclei.
For deuterium for average kinetic energy required tens of keV.
For 1 keV are needed about 10 million degrees temperature.
The huge temperature is done with high power lasers acting hot plasma.
Electromagnetic fields are arranged so that it can maintain hot plasma.
The best results were obtained with the Tokamak-type installations.
ITER: the world's largest Tokamak
Deuterium fuel is delivered in heavy water, D2O.
Tritium is obtained in the laboratory by the following reaction.
Lithium, the third element in Mendeleev's table, is found in nature in sufficient quantities.
The accelerated neutrons which produce the last presented reaction with lithium, appear from the second and the third presented reaction.
Raw materials for fusion are deuterium and lithium.
All fusion reactions shown produce finally energy and He. He is an inert (gas) element. Because of this, fusion reaction is clean, and far superior to nuclear fission.
Hot fusion works with very high temperatures.
In cold fusion, it must accelerate the deuterium nucleus, in linear or circular accelerators. Final energy of accelerated deuterium nuclei should be well calibrated for a positive final yield of fusion reactions (more mergers, than fission).
Electromagnetic fields which maintain the plasma (cold and especially the warm), should be and constrictors (especially at cold fusion), for to press, and more close together the nuclei.
The potential energy with that two protons reject each other, be calculated with the following relationship.
At a keV is necessary a temperature of 10 million 0C.
At 360 keV is necessary a temperature of 3600 million 0C.
In hot fusion one needs a minimum temperature of 3600 million degrees, for a distance between the two particles of 4x10^(-15).
Without a minimum of 4000 million degrees we can't make the hot fusion reaction, to obtain the nuclear power.
Today we have just 150 million degrees made.
To replace the lack of necessary temperature, it uses various tricks.
In cold fusion one must accelerate the deuterium nuclei at an energy of 360 [keV], and then collide them with the cold fusion fuel (heavy water and lithium), and collide them each other.
New Cold Nuclear Fusion
Because obtaining the necessary huge temperature for hot fusion is still difficult, it is time to focus us on cold nuclear fusion.
We need to bomb the fuel with accelerated deuterium nuclei.
The fuel will be made from heavy water and lithium.
The optimal proportion of lithium will be tested.
It would be preferable to keep fuel in the plasma state.
Between deuterium and tritium the smallest radius is the radius of deuterium nucleus.
Where r0=(1,2…1,5).10^-15 the average radius of a nucleon; usual r0=1,45.10^-15
R=r0.(A)^1/3 the average radius of a static nucleus
All these static calculations still refer to a moving particle dynamic. So something is wrong.
For a more precise calculation it is necessary to determine the precise radius of elementary particles in motion.
Based on dynamic hypotheses, solving some difficult and original moving systems of equations, were obtained these three relationships.
First determines particle velocity needed for energy fusion.
Second one calculates the radius of particle on movement.
Third it determines the particle energy to get the cold fusion.
With m0 deuteron=3.34524E-27[kg]
v=1383320.056[m/s] |
RD=3.83566E-19 [m] (dynamic at v=0.004614143c)
U=Ep=3.00674E-10[J]= 1876809963[eV]= 1876809.963[KeV]= 1876.809963[MeV]=1.88[GeV]
Conclusions
Based on dynamic hypotheses, solving some difficult and original moving systems of equations, was obtained a potential energy of 1.88 [GeV] which that the deuteron must to be accelerated and then collided to get the cold nuclear fusion.
Dynamic at high velocity and when the particle mass increase, the particle radius decreases (focuses matter).
Bibliography
- Petrescu F.I., Nuclear Fusion, Alternative Energy Magazine, (2012), available at: https://www.altenergymag.com/content.php?post_type=1931
- "First successful integrated experiment at National Ignition Facility announced". General Physics. PhysOrg.com. October 8, 2010. Retrieved 2010-10-09.
- "Beyond ITER". The ITER Project. Information Services, Princeton Plasma Physics Laboratory. Archived from the original on 7 November 2006. Retrieved 5 February 2011. - Projected fusion power timeline
- Atzeni, Stefano (2009). The Physics of Inertial Fusion. USA: Oxford Science Publications. pp. 12–13. ISBN 978-0-19-956801-7.
- Iiyoshi, A; H. Momota, O Motojima, et al. (October 1993). "Innovative Energy Production in Fusion Reactors". National Institute for Fusion Science NIFS: 2–3. Retrieved 14 February 2012.
- Rolfe, A. C. (1999). "Remote Handling JET Experience". Nuclear Energy 38 (5): 6. ISSN 0140-4067. Retrieved 10 April 2012.
- Heindler and Kernbichler, Proc. 5th Intl. Conf. on Emerging Nuclear Energy Systems, 1989, pp. 177-82. See also Residual radiation from a p–11B reactor
The content & opinions in this article are the author’s and do not necessarily represent the views of AltEnergyMag
Comments (0)
This post does not have any comments. Be the first to leave a comment below.
Featured Product
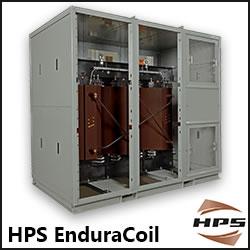