Natural photosynthesis has various steps that are so complex that replicating it is challenging. Each step is equally important in the pathway of converting carbon dioxide and other reactants into energy.
Artificial Photosynthesis: An interesting up and coming alternative energy source
Rajasi Kolhatkar , Dr. Raj Shah, Gavin S Thomas, Mrinaleni Das, Angelina Mae Precilla | Koehler Instrument Company
I. Introduction
In the last decade, there has been a 42% increase in demand [L1] for green energy, or energy produced from sources that do not harm the environment. This high demand for clean, renewable resources has sparked interest in generating energy while minimizing pollution. One way of doing this is through artificial photosynthesis, which aims to artificially replicate the cycle of converting CO2 into oxygen and energy [GT2] using sunlight. Researchers have experimented with many different systems and designs, such as catalytic systems, molecular catalysts, and molecular frameworks, to achieve artificial photosynthesis. [i]
Figure 1. Steps involved in photosynthesis.i[GT3]
Natural photosynthesis has various steps that are so complex that replicating it is challenging. Each step is equally important in the pathway of converting carbon dioxide and other reactants into energy. Figure 1 provides a snapshot of the steps involved in photosynthesis and researchers have focused their efforts on each of these areas:
(a) oxidation of H2O to O2 in the oxygen-evolving center (OEC)
(b) absorption of light by the electron which goes from Photosystem II (PSII) to excited Photosystem II (PSII*).
(c) electron transport chain
(d) another light/photon absorption so the electron goes from PSI to PSI*
(e) cycling of an electron from excited PSI* to PSI and
(f) eventual donation of electrons to Nicotinamide Adenine Dinucleotide Phosphate (NADP+) and the creation of Adenosine Triphosphate (ATP).
The end goal is to try and replicate the natural process of photosynthesis as efficiently as possible so generating energy will be much more sustainable. This review summarizes and evaluates various studies to improve each step described above.
II. Article Research
II.I Artificial Antennas
After Giacomo Ciamician, an Italian chemist, made the first breakthrough in artificial photosynthesis in 1912, others started to realize the great opportunities that could come with possibly being able to generate energy. From this breakthrough, scientists discovered that energy could have the possibility of being artificially harvested. [L8] Balzani et al. concluded that artificial photosynthesis should begin with an artificial antenna to harvest and absorb the light.[L9] [ii] The artificial antenna is a multicomponent system with multiple chromophores (Figure 2a) that can absorb sunlight and convert it to energy. As a way of mimicking how light is absorbed, researchers found that synthetic dyes can be used in place of natural, light-absorbing pigments, such as porphyrins. In plants, these porphyrins are the main chromophores that absorb light and are hence ideally suited as chromophores in antennae. Figure 2 provides examples of light-harvesting antennae, which demonstrates how sunlight gets absorbed into the artificial chromophores to start the energy transfer. [L10] Other examples of artificial antennae include polymers and cyclodextrins. [iii]
More recently, conjugated polymers have shown promise to serve as artificial antennas. They have light capture and emission capabilities, efficient energy transfer, and biocompatibility modifying their functional groups, they can bind directly to the organism and capture light. This provides an advantage over the low absorption efficiency of photosynthetic pigments.[iv]
Figure 2a. Schematic of artificial antenna 2b. Compounds that can serve as light-harvesting antennae.ii
II.II Organic Frameworks
Various research groups have focused on step (a) in Figure 1: the oxidation of H2O to O2 in the oxygen-evolving center (OEC). One method of making this step more efficient, like the natural process, is the formation of heterometallic clusters. These clusters can simultaneously hold the active catalytic sites for CO2 reduction and H2O oxidation half-reactions while maintaining precise cluster structures. The assembly of multiple heterometallic clusters results in heterometallic cluster-based metallic organic frameworks (MOF) (Figure 3). The advantages of MOF are that they improve visible light absorption (progressing to step (b) of Figure 1) and increase CO2 adsorption by increasing the surface area, exposing more active sites to CO2 and H2O. [v]
An increased surface area is also helpful when working with the controlled synthesis of covalent organic framework (COF) multicomponent nanocomposites—another class of promising photocatalysts. In simple terms, these are materials with covalent organic frameworks, which are crystalline porous organic materials at the nanoscale. The increased surface area of these materials, providing ample space for active and binding sites, has led scientists to consider their potential in artificial photosynthesis.
A specific aspect of this research was synthesizing various architectures (Figure 3c), with the yolk-shell structure proving to be the most promising for artificial photosynthesis. COF nanocomposites with a yolk-shell structure have a hollow center, allowing it to accommodate other materials and providing oxidation and reduction sites within one architectural system[GT11] . [vi] With COFs, via heterojunctions, electrons generated by light can move quickly between organic molecules and the semiconductor. This enables efficient transfer of photogenerated electrons, making photocatalysis a lot faster. This exact process helps to simulate the electrons going from PSII to the excited state of PSII*.[vii]
Figure 3a. Core-shell, yolk-shell, and hollow shell architectures of COFs.v
Figure 3b. d) Core shell is not as compact, e) Yolk-shell has more concentrated material in the center, f) hollow shell is more concentrated on the exterior.v
II.III Absorption of Light to Achieve the Excited State
Going back to the previously mentioned Figure 1, the three steps that take place are simple. However, each step has multiple reactions taking place for the pathway to move to the next; this section focuses on step (b) – absorption of light by the electron, which goes from PSII to excited PSII*. [GT12]
To start, carbon dioxide is a tough compound to split because it needs a lot of energy. Photosynthesis is especially difficult as it requires photocatalytic reduction, a process that requires a lot of energy to break the carbon-oxygen bond to form the carbon-hydrogen bond. Achieving photoconversion of carbon dioxide requires highly efficient multielectron and multiphoton processes that can be utilized as catalysts. Two methods are being researched. The first one helps the electron transfer with TiO2, a common photocatalyst known for its high efficiency in multielectron photocatalytic reactions, as shown in Figure 4. This method is helpful and closely simulates the energy absorption observed in the natural process of photosynthesis.[viii] [GT13]
Another method used is scanning tunneling microscopy (STM), which determines the CO2 adsorption and gauges the charge transfers in the reaction. High STM values for CO2 adsorption and charge transfer indicate an improved efficiency in CO2 photoreduction, resulting in an increased charge-separated state. This occurs when the light causes the electrons to move around, creating areas with more positive and negative charges, thereby allowing high electrical conductivity of the photocatalyst.[ix]
Figure 4. Schematic demonstrating the efficiency of TiO2 in multielectron photocatalytic reactions.vii
II.IV ATP
In photosynthesis, catalysts play a critical role in the process because they are used to split the water molecules into a proton gradient that is used to create ATP, an energy molecule. In artificial photosynthesis, photocatalysts are used to accelerate certain reactions that take place. The next few components of research cover step (f) of Figure 1, the creation of ATP, and other valuable products.
The catalyst system developed by the Cao research group utilizes a polymeric carbon nitride-based system that overcame the challenges of poor solubility and mass transport inherent in current CO2 catalysts. The gas-liquid-solid strategy increased the movement of gas molecules and the amount of light absorbed in water. It also improved the efficiency of hydrocarbon production while preventing side reactions. As a result, there was a great increase in the rate of converting CO2 into products that closely resemble the natural process of photosynthesis[GT14] .[x]
II.V Homogeneous -Heterogeneous Hybrid System
A more recent catalytic system that was developed is the homogeneous-heterogeneous hybrid artificial photosynthesis system[GT15] . Researchers have recognized that photocatalysis could be made more efficient and stable, so they have considered a hybrid design. Connecting light-harvesting semiconductors (LHS) and molecular active centers (MAC) reduces the time in which homogeneous and heterogeneous photocatalysts are connected, so energy is harvested much faster.[xi]
Figure 5. Schematic demonstrating hybrid homogeneous-heterogeneous system.x
II.VI Surface-Functionalized Nanoparticles
Another line of research explores the use of surface-functionalized nanoparticles [GT16] as artificial photosynthesis catalysts. Their unique properties are utilized for surface activation of catalysts. Surface activation is the altering of the surface energy chemistry of a certain material to favor a specific property. Modifying a property of the organic molecule can enhance photocatalytic activity by directing certain reaction pathways.[xii] In this case, the chemical bonding of ligands to the catalysts ensures that there are accessible active sites for the catalyst to bind.
One challenge in this research is designing nanocatalysts that can be efficient while also reducing the time of charge transfer from the absorbed light by the nanocatalysts.[xiii] The Lu group of researchers has discussed a promising nanocatalyst, metal halide perovskites (MHPs). They absorb light efficiently and convert it into energy that can be utilized easily and have an efficient charge transfer providing electrons to reduce CO2.[xiv] A reduced charge transfer results in a more efficient process, specifically a faster way of generating ATP or energy. This property is actively sought after in the research field of artificial photosynthesis because it provides a quick and sustainable method of generating energy.
III. Concluding Remarks
The ongoing research on the natural process of photosynthesis has helped further the development of different technologies to make artificial photosynthesis possible. Figure 1 identifies the six key steps that take place in the process. The research to date has focused mainly on steps (a) oxidation of H2O to O2 in the oxygen-evolving center (OEC), (b) absorption of light by the electron which goes from PSII to excited PSII*, and (f) the eventual donation of electrons to the NADP+ and the creation of ATP. These three steps cover the first and last steps of photosynthesis. Over time, the technology that is used for research will become more advanced. Although there has been significant progress in the field of artificial photosynthesis, integrating all the components described in Figure 1 into a working artificial photosynthesis system remains a persistent challenge[GT17] .[L18]
Dr. Raj Shah serves in the role of Director at Koehler Instrument Company in New York, boasting an impressive 28-year tenure with the organization. Recognized as a Fellow by eminent organizations such as IChemE, CMI, STLE, AIC, NLGI, INSTMC, Institute of Physics, The Energy Institute, and The Royal Society of Chemistry, he stands as a distinguished recipient of the ASTM Eagle award. Dr. Shah, a luminary in the field, recently coedited the highly acclaimed "Fuels and Lubricants Handbook," a bestseller that unravels industry insights. Explore the intricacies at ASTM's Long-Awaited Fuels and Lubricants Handbook 2nd Edition Now Available (https://bit.ly/3u2e6GY).
His academic journey includes a doctorate in Chemical Engineering from The Pennsylvania State University, complemented by the title of Fellow from The Chartered Management Institute, London. Dr. Shah holds the esteemed status of a Chartered Scientist with the Science Council, a Chartered Petroleum Engineer with the Energy Institute, and a Chartered Engineer with the Engineering Council, UK. Recently honored as "Eminent Engineer" by Tau Beta Pi, the largest engineering society in the USA, Dr. Shah serves on the Advisory Board of Directors at Farmingdale University (Mechanical Technology), Auburn University (Tribology), SUNY Farmingdale (Engineering Management), and the State University of NY, Stony Brook (Chemical Engineering/Material Science and Engineering).
In tandem with his role as an Adjunct Professor at the State University of New York, Stony Brook, in the Department of Material Science and Chemical Engineering, Dr. Shah's impact spans over three decades in the energy industry, with a prolific portfolio of over 600 publications. Dive deeper into Dr. Raj Shah's journey at https://bit.ly/3QvfaLX.
For further correspondence, reach out to Dr. Shah at rshah@koehlerinstrument.com.
Simultaneously, within the dynamic internship program at Koehler Instrument Company in Holtsville, Rajasi Kolhatkar[1], Gavin S Thomas, Mrinaleni Da, Angelina Mae Precilla[2]
emerge as standout participants. These aspiring talents, all pursuing studies in Chemical Engineering at University of Texas A & M ( Ms. Kolhatkar )and the rest at Stony Brook University, Long Island, NY, thrive under the mentorship of Dr. Raj Shah, the current Chairman of the External Advisory Board of Directors at the Stony Brook university.
[1] Texas A&M University, College Station, TX 77843
[1] Stony Brook University, Stony Brook, NY 11794
i S. Berardi et al., "Molecular artificial photosynthesis," Chemical Society Reviews, 10.1039/C3CS60405E vol. 43, no. 22, pp. 7501-7519, 2014, doi: 10.1039/C3CS60405E.
[1] V. Balzani, A. Credi, and M. Venturi, "Photoprocesses," Current Opinion in Chemical Biology, vol. 1, no. 4, pp. 506-513, 1997/12/01/ 1997, doi: https://doi.org/10.1016/S1367-5931(97)80045-2.
[1] V. Balzani, A. Credi, and M. Venturi, "Photoprocesses," Current Opinion in Chemical Biology, vol. 1, no. 4, pp. 506-513, 1997/12/01/ 1997, doi: https://doi.org/10.1016/S1367-5931(97)80045-2.
[1] X. Zhou, Y. Zeng, F. Lv, H. Bai, and S. Wang, "Organic Semiconductor–Organism Interfaces for Augmenting Natural and Artificial Photosynthesis," Accounts of Chemical Research, vol. 55, no. 2, pp. 156-170, 2022/01/18 2022, doi: 10.1021/acs.accounts.1c00580.
[1] L.-Z. Dong et al., "Stable Heterometallic Cluster-Based Organic Framework Catalysts for Artificial Photosynthesis," Angewandte Chemie International Edition, vol. 59, no. 7, pp. 2659-2663, 2020, doi: https://doi.org/10.1002/anie.201913284.
[1] M. Zhang et al., "Controllable Synthesis of COFs-Based Multicomponent Nanocomposites from Core-Shell to Yolk-Shell and Hollow-Sphere Structure for Artificial Photosynthesis," Advanced Materials, vol. 33, no. 48, p. 2105002, 2021, doi: https://doi.org/10.1002/adma.202105002.
[1] M. Zhang et al., "Semiconductor/Covalent-Organic-Framework Z-Scheme Heterojunctions for Artificial Photosynthesis," Angewandte Chemie International Edition, vol. 59, no. 16, pp. 6500-6506, 2020, doi: https://doi.org/10.1002/anie.202000929.
[1] D. Li et al., "Accelerating Electron-Transfer Dynamics by TiO2-Immobilized Reversible Single-Atom Copper for Enhanced Artificial Photosynthesis of Urea," Advanced Materials, vol. 34, no. 51, p. 2207793, 2022, doi: https://doi.org/10.1002/adma.202207793.
[1] W. Tu, Y. Zhou, and Z. Zou, "Photocatalytic Conversion of CO2 into Renewable Hydrocarbon Fuels: State-of-the-Art Accomplishment, Challenges, and Prospects," Advanced Materials, vol. 26, no. 27, pp. 4607-4626, 2014, doi: https://doi.org/10.1002/adma.201400087.
[1] Y. Xia et al., "Improving Artificial Photosynthesis over Carbon Nitride by Gas–Liquid–Solid Interface Management for Full Light-Induced CO2 Reduction to C1 and C2 Fuels and O2," ChemSusChem, vol. 13, no. 7, pp. 1730-1734, 2020, doi: https://doi.org/10https://doi.org/10.1002/cssc.201903515.1002/cssc.201903515.
[1] Z. Jiang et al., "Homogeneous–Heterogeneous Hybrid Artificial Photosynthesis Induced by Organic Semiconductors with Controlled Surface Architectures," Advanced Functional Materials, vol. 33, no. 34, p. 2303335, 2023, doi: https://doi.org/10.1002/adfm.202303335.
[1] B.-H. Lee et al., "Reversible and cooperative photoactivation of single-atom Cu/TiO2 photocatalysts," Nature Materials, vol. 18, no. 6, pp. 620-626, 2019/06/01 2019, doi: 10.1038/s41563-019-0344-1.
[1] G. Martí et al., "Surface-Functionalized Nanoparticles as Catalysts for Artificial Photosynthesis," Advanced Energy Materials, vol. 13, no. 21, p. 2300282, 2023, doi: https://doi.org/10.1002/aenm.202300282.
[1] K. Su, S.-X. Yuan, L.-Y. Wu, Z.-L. Liu, M. Zhang, and T.-B. Lu, "Nanoscale Janus Z-Scheme Heterojunction for Boosting Artificial Photosynthesis," Small, vol. 19, no. 32, p. 2301192, 2023, doi: https://doi.org/10.1002/smll.202301192.
The content & opinions in this article are the author’s and do not necessarily represent the views of AltEnergyMag
Comments (0)
This post does not have any comments. Be the first to leave a comment below.
Featured Product
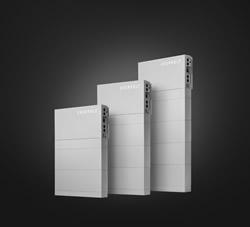