NMR spectroscopy facilitates advancement in solar energy cells
As the climate crisis deepens, it is becoming ever more urgent to find alternatives to the energy sources we are presently dependent on. Solar energy has proved to be one of the most significant alternatives to fossil fuels; both abundant and renewable, it is a beneficial energy source in countries which currently rely on electricity – but could also significantly change the lives of the 800,000,000 people worldwide without any access to electricity.[i] According to the United Nations, about 29 percent of electricity currently comes from renewable sources – so there is clearly the potential to expand.[ii]
However, solar cells (SCs) made chiefly of silicon – comprising over 90 percent of cells produced[iii] – require energy-intensive manufacturing processes. Their fabrication involves the need for very high temperatures (~1400 °C), they have the potential to leak harmful materials into the environment, and few are recycled, meaning they contribute to growing landfill.[iv] In the last 15 years, halide-based perovskite solar cells (PSCs) have been developed as an appealing alternative due to their excellent power conversion efficiency,[v] but requiring much milder manufacturing conditions and being much more lightweight compared to their silicon counterparts.
The research at the University of Birmingham’s School of Chemistry focuses on the development of new solar cell materials. The team uses solid-state nuclear magnetic resonance (NMR) spectroscopy and a range of other characterization techniques to focus on promising structures for optoelectronics, specifically to understand what causes the comparatively rapid degradation of halide-based PSCs. The aim is to improve society’s access to solar power and thus to electricity, minimizing the environmental impact of alternative energy sources.
Diversifying energy sources
There is a pressing need to develop and improve solar cell technology to ensure it remains both effective and efficient, while ensuring it is environmentally friendly. Halide-based PSCs – developed as an alternative to silicon SCs – while economically sound, tend to be more susceptible to moisture, temperature, light, and air, leading to a more rapid rate of degradation.[vi] While PSCs have improved in efficiency over the last decade, they still only guarantee about one year of operation in comparison to the 25 years offered by silicon SCs.[vii] The objective is not to replace silicon SCs but rather to complement them and diversify the ways in which we can produce solar energy – researchers believe it is among the key strategies that can substantially reduce our reliance on fossil fuels. One of the most efficient ways to convert solar energy to electricity is in so-called multijunction devices, where silicon and perovskites are combined to harvest energy across the solar spectrum more effectively.
Analyzing halide perovskites has previously posed a challenge, due to their complex chemical composition. It has been shown that chemical degradation products are easily formed on the surface of the polycrystalline perovskites because of their soft ionic nature,[viii] but it has been more difficult to isolate their structure and individual impact on device stability at the atomic level.[ix] Understanding the nature of degradation is essential if we are to develop better solar cells with improved stability and lifespan.
Atomic-level insight
Various spectroscopic techniques have previously been used for the analysis and characterization of perovskites, such as Raman spectroscopy, Fourier transform infrared (FTIR) spectroscopy, and photoluminescence spectroscopy, in addition to a variety of microscopic methods. However, the complex mixture of chemical elements and additives within the PSCs make their analysis a challenging task, and optical spectroscopies and X-ray diffraction often fall short in providing answers to the behavior of individual chemical components within functioning solar cells.
NMR, in contrast, offers quantitative insights on specific isotopes that help the researchers understand the behavior of each component within a functioning cell. The use of in situ NMR allows element-specific monitoring of real-time degradation in functioning solar cells – such as that caused by light, humidity, and ambient air – by studying the dynamics and mobility of ions within the perovskite structure. This is essential for understanding charge transport processes, ion migration, and other factors that influence the efficiency and stability of the solar cell. Their use of mechanosynthesis enables the synthesis of large libraries of materials with diverse structures and properties, while examining specific nuclei within a material’s structure using solid-state NMR.
When making new halide PSCs, for example, the team introduces very dilute additives in the order of 1 percent relative to the perovskite, which are impossible to identify using diffraction techniques alone. Combining the insights of diffraction techniques with structural information from NMR analysis, which allows access to the atomic level, leads to structure-property relationships that provide a more comprehensive picture of the material.
Combining NMR with a variety of synthetic strategies, diffraction and optical spectroscopies allows an understanding of the materials needed to make more sustainable solar cells. An exciting strategy is mechanosynthesis, a green protocol that makes the synthesis of large libraries of compounds with diverse properties and structures very straightforward.
One area of research involves compositional engineering of fully inorganic halide perovskites.[x] For example, it was discovered that the partial replacement of lead with europium can enhance the stability of the perovskite lattice,[xi] and that barium provides a passivation layer around the grain boundaries of the films leading to enhanced efficiency.[xii] Their group also actively works on engineering organic-inorganic (hybrid) perovskite materials. This combining of organic and inorganic components has seen the photovoltaic performance of hybrid PSCs rise to 25 percent from less than 4 percent in the last ten years.[xiii]
Future capabilities of solid-state NMR
In collaboration with the team of Prof. Ted Sargent from Northwestern University, the team has been contributing to the development of triple-junction solar cells, in which different solar cell absorbers are stacked on top of each other.[xiv] Each stack is made from a semi-conductor of different bandgap energy which allows the cells to harvest different sections of the solar spectrum more efficiently.[xv] Solid-state NMR has played a critical role in understanding why these stacked compositions work so effectively and how to prevent them from operational instabilities.
Collaborative research at the Swiss Federal Institute of Technology Lausanne (EPFL) is trying to push the capabilities of solid-state NMR even further through the use of dynamic nuclear polarization (DNP) with magic-angle spinning (MAS) to improve the selectivity of NMR analysis. Prior to this research, MAS DNP had not previously been applied specifically to halide perovskites. This proves not only that it is possible, but also that it leads to significant enhancements in sensitivity and can reduce experiment time from several months to only a few hours.[xvi]
More specifically, two DNP methods were compared to analyze cesium lead chloride: metal-ion DNP, which incorporates paramagnetic metal ions (Mn2+) into the perovskite structure, and incipient wetness impregnation (IWI) DNP, which involves using an organic biradical solution to coat the particles of the solid. By looking at factors such as particle size, dopant concentration, relaxation times and how the material’s surface interacts with liquids, it was found that metal-ion DNP is highly effective for obtaining detailed structural information on the material’s bulk, where the IWI DNP works best for studying the material’s surface.
In the future, DNP NMR techniques will be invaluable in determining atomic-level structural information of mass-limited perovskite samples, including thin-film devices, which are the most industrially relevant form of perovskites that needs to be studied.
Designing more environmentally friendly solar cells
The growing severity of the climate crisis is driving a demand for a substitution to fossil fuel-based energy sources and has also uncovered a need for more environmentally friendly solar cells which have lower environmental impact during their manufacture, deployment and disposal. Halide-based PSCs, an alternative to silicon based solar cells, require milder manufacturing conditions and substantially less photo absorbers, this in turn makes them much more lightweight and easier to transport. The challenge is that halide perovskites degrade more rapidly than silicon – but solid-state NMR is helping uncover the processes via which this occurs and discover a way of designing solar cells with a longer lifespan.
Ongoing halide PSC research could lead to better efficiency, lowered costs, a reduction in the use of toxic materials and improvements to stability and performance.[xvii] Halide PSCs currently have a lifespan of about one year, but by harnessing the power of solid-state NMR to discover and stabilize new materials, the goal is to increase this to a decade or more.
Combining DNP with MAS will allow further expansion of the capabilities of solid-state NMR, uncovering discoveries for optoelectronic applications. Ultimately, longer lasting solar cells will allow us to make better use of solar energy, something which will not only minimize our dependence on non-renewables but could also change the lives of millions of people worldwide, who for reasons such as cost, location, and practicality, do not currently have access to electricity.
About the author
Dominik J. Kubicki is an Assistant Professor in the School of Chemistry at the University of Birmingham, UK, and a Visiting Professor in the Department of Physics at the University of Warwick. He graduated from the Warsaw University of Technology, Poland in 2013 and completed his PhD at EPFL, Switzerland in 2018. He held a Marie Curie-Skłodowska Fellowship at the University of Cambridge, UK from 2018 to 2021. In 2022, he received an ERC Starting Grant underwritten by UK Research and Innovation to develop new atomic-level strategies to study perovskite solar cells. His research focuses on new materials for sustainable optoelectronic technologies and benefits from the unique capabilities of the UK High-Field Solid-State NMR Facility at the University of Warwick.
References:
[i] IEA. SDG7: Data and Projection. IEA. 2023. Available at: https://www.iea.org/reports/sdg7-data-and-projections/access-to-electricity. Last accessed: Oct 29, 2023.
[ii] United Nations: Powering a safer future. https://www.un.org/en/climatechange/raising-ambition/renewable-energy. Last accessed December 15, 2023.
[iii] Crownhart, C. Solar panels are a pain to recycle. MIT Technology Review. 2021. Available at: https://www.technologyreview.com/2021/08/19/1032215/solar-panels-recycling/. Accessed October 29, 2023.
[iv] Tsuo YS et al. Environmentally benign silicon solar cell manufacturing. NREL. 1998. Available at https://www.nrel.gov/docs/legosti/fy98/23902.pdf. Accessed: Oct 29, 2023.
[v] Contreras-Bernal L et al. Impedance analysis of perovskite solar cells: a case study. J Mater Chem A. 2019; 7, 12191-12200. DOI: 10.1039/C9TA02808K.
[vi] Dahlman CJ et al. Interfaces in metal halide perovskites probed by solid-state NMR spectroscopy. J Mater Chem. 2021; 9, 19206-19244. DOI: 10.1039/D1TA03572J.
[vii] Alanazi AQ et al. Benzylammonium-mediated formamidinium lead iodide perovskite phase stabilization for photovoltaics. Adv Funct Mater. 2021; 31, 30 (2101163).
[viii] Xu T et al. Simultaneous lattice engineering and defect control via cadmium incorporation for high-performance inorganic perovskite solar cells. Adv Sci. 2022; 9, 36 (2204486). DOI: 10.1002/advs.202204486.
[ix] Xie H et al. Decoupling the effects of defects on efficiency and stability through phosphonates in stable halide perovskite solar cells. Joule 5. 2012; 1246–1266. DOI: 10.1016/j/joule.2021/04.003
[x] Xu T et al. Simultaneous lattice engineering and defect control via cadmium incorporation for high-performance inorganic perovskite solar cells. Adv Sci. 2022; 9, 36 (2204486). DOI: 10.1002/advs.202204486.
[xi] Xiang W, Wang Z, Kubicki DJ, et al. Europium-doped CsPbI2Br for stable and highly efficient inorganic perovskite solar cells. Joule. 2019;3(1):205-214. doi:10.1016/j.joule.2018.10.008.
[xii] Xiang W, Wang Z, Kubicki DJ, et al. Ba-induced phase segregation and band gap reduction in mixed-halide inorganic perovskite solar cells. Nature Communications. 2019;10(1). doi:10.1038/s41467-019-12678-5.
[xiii] Xu T et al. Simultaneous lattice engineering and defect control via cadmium incorporation for high-performance inorganic perovskite solar cells. Adv Sci. 2022; 9, 36 (2204486). DOI: 10.1002/advs.202204486.
[xiv] Wang Z, Zeng L, Zhu T, et al. Suppressed phase segregation for triple-junction perovskite solar cells. Nature. 2023;618(7963):74-79. doi:10.1038/s41586-023-06006-7.
[xv] Corkish, R. Tandem and multijunction III-V cells. Elsevier Science. 2004. Extract available at: https://www.sciencedirect.com/topics/engineering/tandem-cell. Last accessed Oct 29, 2023.
[xvi] Mishra, A et al. Dynamic nuclear polarization of inorganic halide perovskites. J Phys Chem C. 2023; 127, 23, 11094-11102. DOI: 10.1021/acs.jpcc.3c01527.
[xvii] Tiarnan A. S. Doherty et al., Stabilized tilted-octahedra halide perovskites inhibit local formation of performance-limiting phases. Science374,1598-1605(2021). DOI: 10.1126/science.abl4890.
Comments (0)
This post does not have any comments. Be the first to leave a comment below.
Featured Product
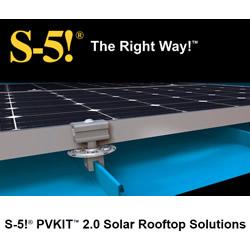