Any time a large amount of energy is squeezed into a tight space, there is a risk that it will escape in an uncontrolled manner. When this happens, fire is a common result and explosions are possible.
Fire Suppression in Battery Energy Storage Systems

Article from | Stat-X® Condensed Aerosol Fire Suppression
What is a battery energy storage system?
A battery energy storage system (BESS) is well defined by its name. It is a means for storing electricity in a system of batteries for later use. As a system, BESSs are typically a collection of battery modules and load management equipment. BESS installations can range from residential-sized systems up to large arrays of BESS containers supporting a utility-grade wind farm or grid services.
BESSs are installed for a variety of purposes. One popular application is the storage of excess power production from renewable energy sources. During periods of low renewable energy production, the power stored in the BESS can be brought online.
Two common types of BESSs are lead-acid battery and lithium-ion battery types. Both essentially serve the same purpose. However, approximately 90% of BESS systems today are of the lithium-ion variety. Lithium-ion batteries are so well adopted because they provide a high energy density in a small, lightweight package and require little maintenance.
Lithium-ion batteries contain a positive cathode and a negative anode. Lithium ions move from the negative anode to the positive anode during discharge and back when charging. This mechanism is immersed in an ion-conducting electrolyte. The electrolyte is a low-viscosity flammable liquid solvent.
Taken together in a housing or container, the lithium-ion batteries are called “cells.” A BESS can contain dozens, hundreds, or even thousands of cells to store energy. The cells are typically packed in modules held in racks, and the racks are normally stored in shipping-container-type structures. Obviously, residential models are much smaller and are often installed in a home garage or basement.
What is the fire risk with a lithium-ion BESS?
Any time a large amount of energy is squeezed into a tight space, there is a risk that it will escape in an uncontrolled manner. When this happens, fire is a common result and explosions are possible.
Several recent incidents in large BESS installations demonstrate how sizable BESS fires can be, how difficult they are to extinguish, and how they can be dangerous to first responders.
Arizona Public Service (Surprise, Arizona) – April 19, 2019
Arizona Public Service operates a large-scale BESS at their solar array site in Surprise, Arizona. Smoke was observed coming from a lithium-ion BESS container. The fire department was called and arrived on scene.
Approximately three hours after arrival, fire crews opened the doors to the still-smoking container. When fresh air mixed with the flammable vapors inside the container, an explosion occurred. Four firefighters were injured.
Tesla (Moorabool, Victoria, Australia) – July 30, 2021
Tesla’s 300 MW ”big battery” project suffered a catastrophic fire that burned for four days. Reputedly the largest such BESS fire in the world to date, the local fire service experienced significant challenges in extinguishment. They eventually cooled surrounding structures and allowed the fire to burn out.
Private Operator (Seoul, South Korea)– April 6, 2021
A BESS installed at a private solar farm caught fire and burned for hours. The fire destroyed 140 batteries, did structural damage to the plant, and burned seven power generation modules. There were no injuries, but the fire did over $300,000 in damage.
While all of these incidents had large direct fire losses, in many cases the indirect costs can be far higher. Downtime, lost productivity, and harm to the company’s image can quickly exceed the loss of the damaged equipment.
What causes fires in BESSs?
To understand the fire problem for BESSs, it is important to grasp how they fail. Their mode of failure illustrates how fire (and/or explosion) is the end result of a multi-step process. Understanding this process identifies opportunities where an intervention can be introduced to avert a disaster.
There are four stages or phases of battery failure:
-
Stage One: Battery Compromise
​Batteries may be damaged or otherwise compromised in one of four ways:-
Thermal – Heat stress from internal overheating or external heating from a fire or strong heat source.
-
Electrical – The battery is overcharged, or a short circuit develops.
-
Mechanical – The battery container is damaged in some fashion.
-
Manufacturing Defect – A problem in manufacturing leads to a thermal or electrical failure event.
-
-
Stage Two: Off-Gassing
Once the battery is compromised, the internal temperature and pressure of the battery increases due to a build-up of gases. The integrity of the battery container fails, and the gases are released. The gases are mostly vaporized electrolyte which is flammable or explosive. Off-gassing can occur for as little as two minutes to almost thirty minutes before the next stage.
-
Stage Three: Smoke Production
As the battery fails, the voltage drops to zero, and the anode and cathode short circuit. With all the battery’s stored energy flowing through the short, the temperature of the battery will quickly spike, to over 300°C. This causes smoke to be produced from inside of the battery. Smoke production is the first step in thermal runaway and can become combustible.
Thermal runaway is a chain reaction in which:
- The exothermic reaction of a failing battery cell overheats an adjacent battery cell or module.
-
Stage Four: Fire
Fire can ensue rapidly after the evolution of smoke. Or the thermal runaway event can continue for hours without any flame production. During this period, large quantities of flammable vapors and gases are being produced and contained in the enclosure creating an explosive atmosphere.
In many instances, however, ignition occurs, and a fire develops inside of the BESS enclosure. As the battery components are consumed by fire, gases—such as carbon dioxide, carbon monoxide, hydrogen, and unburned hydrocarbons—can also collect in the enclosure. This creates a flammable mixture that has a collective flashpoint much lower than the flashpoints of the individual gases.
Fire inside the enclosure can cause or escalate the speed of a thermal runaway, leading to a devastating and difficult-to-extinguish event.
What types of interventions can be used in a BESS event?
With any type of incident where mitigation is possible, it always best to intervene at the earliest possible stage. As was described in the stages of battery failure, there are opportunities to take early action to avert a disastrous outcome.
BESSs can be protected by the following systems:
Battery Management System
The simplest and earliest intervention is effective battery management. A battery management system’s (BMS) main role is to prevent damage to the battery cells from over-charging and over-discharging. The BMS also:
-
Calculates the charge remaining on the battery
-
Monitors the temperature of the battery
-
Monitors for shorts and faulty connections
-
Maintains the charge within the cells in the optimal performance range
If the BMS detects any abnormal conditions, it shuts the battery down. This protects the cells from damage. Most people have witnessed this when cell phones and laptops suddenly die with no warning. This is because the BMS sensed the charge remaining was outside of its operating threshold and shut the battery down.
A BMS provides two important services to the end user. First, it extends the life of the battery by keeping it in the optimum operating condition. And most importantly, the BMS can act to shut a battery down before it reaches a point where it becomes a safety hazard.
Gas Detection
By and large, BMSs do their job extremely well. However, if the BMS becomes damaged or there is a manufacturing defect, the battery can become unstable and begin to fail.
As discussed previously, as the unstable condition of the battery escalates, the temperature and pressure inside the cell begin to rise as flammable gases accumulate. At some point, either an installed vent opens or there is failure in the battery housing (typically a pouch or shell type), and the gases escape.
Research was conducted on lithium-ion cells where they were tested to failure. Gas chromatography of the off gas revealed that the primary constituent was ethyl methyl carbonate which is the electrolyte inside the cell.
Ethyl methyl carbonate is considered a Class II flammable liquid. It has a flashpoint of approximately 80°F. Once vaporized by the exothermic reaction that occurs during failure, it is highly flammable.
Off-gassing can occur for up to thirty minutes before smoke is produced. Gas detection offers the first chance to intervene after the BMS fails. Gas detection provides far quicker notification of the problem than does a smoke, heat, or flame detector. With gas detection, this is an opportunity to mitigate the problem before it requires an active response action from fire suppression equipment.
When the gas detector alerts to the presence of an off gas, it can activate several mitigating actions. Perhaps the most important is shutting down power to the affected cell(s). Additionally, the gas detection equipment can:
-
Activate a ventilation system within the BESS enclosure to remove flammable gases and heat
-
Activate local and remote alarms
-
Provide an early warning for operators to take additional measures
Fire Suppression
Fire suppression is the last line of defense. The discharge of agent means that all other interventions have failed. However, the nature in which batteries fail and their very design make total extinguishment challenging.
After gas detection, the next opportunity for fire detection is by the detection of smoke. In this instance, a smoke detector alarms, and the signal triggers a fire suppression system that activates.
But as we mentioned and was highlighted at the Arizona Public Service (APS) explosion, thermal runaway that produces smoke can occur and slowly build up for hours. At the APS incident, the smoke detection system operated as designed and activated a clean-agent fire suppression system.
However, even after discharge of the clean agent, thermal runaway continued. For three hours before the fire crews opened the container doors (initiating an explosion), large quantities of flammable smoke continued to be produced.
So, what went wrong at the APS incident? From a fire suppression agent perspective, the chosen clean agent system utilizing a fluoroketone agent, was not designed correctly. The system did operate and discharged a 10% concentration of agent.
According to an investigative report produced by DNV GL (now known as DNV), an international leader in battery testing, “The {fluoroketone} clean agent was inadequate and inappropriate to stop or prevent cascading thermal runaway of multiple battery cells or modules.”
The report went on to cite the agent manufacturer where they stated in comments to a draft of NFPA 855 Standard for the Installation of Stationary Energy Storage Systems®: “Clean agents are demonstrably ineffective in preventing and stopping thermal runaway, as are foam and dry chemical.”
Since the clean agent was designed for extinguishing incipient fires, it was unsuccessful at stopping the non-flaming thermal runaway. Thirty minutes after discharge, there was no agent in suspension and the space was completely vulnerable to explosion and fire. The failure to use an appropriately designed fire suppression system including failure to completely seal the enclosure thus allowing early depletion of agent concentration and reduced hold time was cited as one of the primary contributing factors to the severity of the incident.
With clean agents being ineffective for BESS incidents, then what about using a sprinkler system? Traditional sprinklers are not a good choice. The confined nature of battery cells effectively prevents the water from reaching the heated area. Sprinkler systems also require a dedicated water supply which can be problematic in many areas. Lastly, the water discharge can damage the BESS components and raise environmental concerns due to water runoff.
To provide superior fire protection for BESSs, a specialized agent is required. The ideal agent in this case is one that will:
-
Limit propagation of thermal runaway
-
Suppress any fire present
-
Limit damage to components
-
Does not require significant infrastructure
Despite the difficult nature of providing these “must haves,” there is an agent that does exactly this. The Stat-X® condensed aerosol fire suppression system is the ideal agent for BESS fire suppression.
Stat-X has been tested extensively, resulting in verification of its performance in these categories. First, Fireaway contracted DNV—the same organization that investigated the APS incident—to conduct trials of Stat-X on lithium-ion battery cell fires. The results were extremely positive:
-
Stat-X was proven effective at extinguishing single- and double-cell lithium-ion battery fires.
-
Residual Stat-X airborne aerosol in the hazard provides additional extended protection against reflash of the fire.
-
Stat-X reduced oxygen in an enclosed environment during a battery fire to 18%.
-
The residence time of gases and aerosols during Stat-X deployment is a function of when the atmosphere is ventilated.
Second, Fireaway contracted PVEL, a leader in renewable energy product and regulatory testing, to perform full-scale testing in compliance with UL 9540a. These results were also positive. During the period of Stat-X discharge and agent retention time:
-
Thermal runaway did not propagate to adjacent battery racks
-
No flames were observed
-
No significant increase in temperature was observed
-
No deflagration or explosion was observed
The Stat-X total flooding system offers the unique combination of limiting thermal runaway propagation while also suppressing and knocking down the fire. The self-contained units can be connected to any type of detection system or can be specified to activate at a pre-determined temperature or gas concentration level. And the virtually maintenance-free and compact units require no piping or other infrastructure requirements.
It is also important to note that condensed aerosol fire suppression agents have no global warming or ozone depletion properties. The agent is rated for normally occupied areas.
What is a best practice for protecting BESSs?
Adequately protecting a BESS requires a complete and integrated system. Each component has its place and functions to provide layered protection. A highly protected BESS might look like this:
-
A well-designed and manufactured battery management system provides oversight of the battery’s functions and operating conditions.
-
A gas detection system is employed to shut down faulty cells and:
-
Activate a ventilation system
-
Sound local and remote alarms
-
-
A total flooding condensed aerosol fire suppression system is installed and connected to the fire detection system.
To aid in first responder safety, the following can help prevent an incident such as the APS explosion:
-
A fire department quick connect dry pipe sprinkler or water mist system so fire crews can cool the interior of the enclosure.
-
Thermal imaging cameras for detecting heat levels and hot spots from outside of the enclosure.
Stat-X® photo courtesy of Fireaway Inc.
Lithium-ion BESSs present a clear risk of fire and explosion. Their design and mode of failure make many traditional fire suppression agents and tactics ineffective. To adequately protect BESSs, a system of layered protection is required to prevent the BESS from experiencing a severe thermal runaway event. If these measures are unsuccessful, a fire suppression agent such as Stat-X is required to quickly suppress a fire, limit propagation of the thermal runaway, and maintain total flood protection to suppress reflash.
The content & opinions in this article are the author’s and do not necessarily represent the views of AltEnergyMag
Comments (0)
This post does not have any comments. Be the first to leave a comment below.
Featured Product
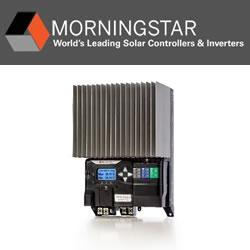