Despite the positive characteristics of SMRs, the high costs and dangers of radioactive material deprioritize the technology compared to traditional and reliable energy sources like fossil fuels.
Recent Advancements in Small Modular Reactor (SMR) technology
Dr. Raj Shah and Bradford Leung | Koehler Instrument Company
Introduction
The International Atomic Energy Agency defines small modular reactors (SMRs) as nuclear reactors capable of generating up to 300 megawatts of electricity (MWe) per unit. Unlike traditional large-scale nuclear reactors that exceed 1,000 MWe, SMRs focus on modularity and flexibility of deployment, allowing for wider adaptability. Today, the most widely used nuclear reactors are pressurized water reactors (PWRs). PWRs employ pressurized water as both a coolant and a moderator to transfer heat from the reactor core to a secondary water circuit, where steam is then produced to drive turbines or power thermoelectric generators [1].
In the face of the pollution and climate change that plagues modern society, SMRs address the growing demand for renewable energy by providing a low-carbon alternative to traditional power generation. Despite the positive characteristics of SMRs, the high costs and dangers of radioactive material deprioritize the technology compared to traditional and reliable energy sources like fossil fuels. As the support of SMRs is driven from those factors, recent advances have prioritized making SMRs safer, more accessible, economically viable, and suitable for diverse applications.
Economic and Environmental Viability
In 2018, the U.S. Department of Energy allocated 900 million dollars to fund domestic SMRs as part of their commitment to clean energy. The U.S. Secretary of Energy, Jennifer M. Granholm, emphasized that this investment "advances environmental protection and community benefits, creates new, good-paying, high-quality jobs, and reinforces America’s leadership in the nuclear industry” [2].
The primary benefit of SMRs is their utilization of clean nuclear energy for electricity generation, as nuclear reactors do not produce greenhouse gases or carbon emissions. The Cambridge University Press [3] estimates a carbon emission reduction of 65% to 90% across all sectors will limit the global temperature increase to 1.5oC by 2050 relative to emission trends from 2010. However, widespread appeal and implementation of these technologies is hindered by economic barriers within the market.
Figure 1: “Economically viable deployment stacks of SMRs at industrial facility processes in SPP (left) and ERCOT (right) when providing process heat only across avoided natural gas costs” [4].
In 2023, Vanatta et al. [4] associated with the University of Michigan compared the markets of the Electric Reliability Council of Texas (ERCOT) with the Southwestern Power Pool (SPP) to assess SMRs’ impact when substituting petroleum fuels. Researchers first analyzed the energy demand from industries in these two markets and the current initial costs of various types of SMRs. Comparing the different types, they concluded that SMRs were not cost-competitive when gas prices were lower than $8 per MMBtu, as seen in Figure 1. The availability of petroleum-based fuels coupled with lax government regulations surrounding competitive alternative energy markets far outweighs the costs of SMRs. To justify the switch to clean emissions, the overall cost of SMR systems on the market must be researched and reduced.
Recent Designs, Their Modularity and Safety
NuScale Power Corporation, an American SMR marketing company based in Oregon, is the first company to have their SMR design approved by the U.S. Nuclear Regulatory Commission (NRC) in 2022. Their NuScale US600 SMR prioritizes in-house production and modular designs, powering planes with up to twelve reactors that output 50 MWe each, depicted in Figure 2. A notable feature of the NuScale design is its advanced passive safety mechanisms, including a gravity-driven boron dilution system that regulates reactor output without relying on external power and ensures reliable fail-safe measures during emergencies.
Figure 2: NuScale US600 SMR Diagram, U.S. NRC, 2022 [5].
Another NRC-approved SMR design originates from Holtec International, an American energy equipment supplier company in New Jersey. The Holtec SMR-300 design incorporates a single integrated-pressurized-water-reactor (iPWR) design to generate up to 300 MWe. Although the reactors themselves are not modular, the designs of its coolant pumps and steam generators offer this characteristic, as shown in Figure 3. Holtec’s design also incorporates gravity-driven safety systems that can utilize both water- and air-cooling systems. However, the effectiveness of the design raises environmental concerns due to its high rate of thermal pollution caused by the heating of natural bodies of water.
Figure 3: Holtec SMR-300 Design, U.S. NRC, 2021 [5].
Figure 4: Energy Multiplier Module EM2, U.S.NRC, 2021 [5].
Finally, the Energy Multiplier Module (EM2) design by General Atomics, an American transportational technology developer located in California, is the only pre-approved SMR that does not incorporate any PWR designs. While many PWR designs are limited by the thermal properties of water the EM2 reactor uses a chemically inert helium gas cooling system to circumvent these drawbacks and generate power through a closed-cycle gas turbine, shown in Figure 4. The design also offers unique underground and remote settings for safety and fuel control. General Electric's EM2 design features passive decay heat removal, enabling reactor cooling in potential shutdown scenarios through highly conductive materials and naturally circulating helium, reducing the need for electric mechanisms. Additionally, the design also implements ceramic-coated uranium carbide fuel with high melting points to regulate heat, operating at a high ~850°C for burning waste and greater power generation compared to the average PWR at ~300°C. However, the unconventional usage of inert gas for a cooling system causes concern for the transportation and storage of helium. In addition, its high operating temperatures raise regulatory hurdles and keep this model in its conceptual stages [5].
Alternative Fuel Cells
Recent SMR developments have also explored new fuel sources and technologies that offer greater economic sustainability compared to current reactor designs. Since the cold war most of the highly enriched uranium (HEU) is designated as weapon material. This HEU, classified by its minimum 20% weight of radioactive uranium-235, undergoes a lower enrichment process to create high-assay-low-enriched Uranium (HALEU), which is more suitable as a reactor fuel. HALEU cores are the foundation of modern SMRs and PWR designs. Current research focuses on the development of compounds in tandem with HALEU cores as well as the production of different radioactive fuels and non-PWR designs with the potential to improve the longevity and efficiency of SMR applications.
For instance, the previously mentioned EM2 SMR utilizes a ceramic-coated fuel cell for higher heat retention and tolerance, and an alternative helium gas-cooled core than the usual PWR design. The differences from traditional SMR PWR designs address its main issues of thermal pollution and difficulty in controlling the physical properties of water. All in all, the EM2 is one of many SMR utilize promising fuels and systems that while primitive will develop with time and research.
Figure 5: MDBAP Design [6].
Another area of exploration in SMRs is the improvement of existing technologies such as absorber pins. The most used materials range from boron, hafnium, and cadmium, which are chosen to absorb neutrons and control overall radioactivity and regulate operational temperatures in PWRS. In 2024, Bolukbasi et al., [6] a group associated with Bangor University in the United Kingdom, published a study exploring the use of 7% enriched HALEU on experimental absorber pins. In Figure 5, the Moderated Discrete Burnable Absorber Pins' (MDBAPs) design features zirconium diboride (ZrB₂) and uranium diboride (UB₂) absorber pins with a commonly used beryllium oxide moderator pin. Unlike the traditional composition of absorber pins, the experimental pins exhibit a higher affinity to the HALEU reactor core and moderation properties, meaning its capacity to absorb and regulate fission is larger. A Studsvik CASMO-4/SIMULATE-3 software was implemented alongside the pins to conduct fuel cycle simulations. Their main goal was to simulate a 36-month fuel cycle, twice as long as the industrial standard of 18 months. From the simulations, the new absorber pins showed an increase in fuel capacity throughout the 36-month cycle. The experimental absorber pins increased both burnup (energy produced per unit of fuel) and cycle length, which lengthened fuel capacity - overall demonstrating effectiveness and promising future of the uranium and zirconium diboride pins. While these results are significant, widespread implementation is still far from viable due to a multitude of uncertainties within the experimental fuel composition. Primarily, 7% enriched HALEU utilized in the simulation is a much higher concentration than the commonly implemented enrichment levels of 3-5% in SMRs. Additionally, a 36-month fuel cycle opposed to the traditional 18-month cycle is unorthodox and riskier for investors. These factors, coupled with the lack of physical results, create problems with the otherwise promising fuel cells.
Figure 6: Variation of burnable absorber concentration with burnup for the investigated fuel types [7].
Furthermore, molten salt thorium has also surfaced as a promising cost-effective alternative. In 2024, Amatullah et al., [7] a research team at the Bandung Institute of Technology in Indonesia, explored thorium-based energy cells, specifically dual fuel systems of uranium and thorium, in an integral-pressurized-water-reactor. Thorium, compared to uranium, is more accessible and can produce larger amounts of radioactive energy than its counterpart. The research was justified through these facts with thorium’s higher accessibility, reactivity, conversion ratios, and neutron multiplication factors which result in larger outputs of energy. In contrast to HALEU, thorium-based fuels are challenged by high power peaking and fertile nature, therefore thorium fuels require a mechanism for fission initiation by uranium isotopes. As a result, parts of the fuel where initiation occurs are subject to localized burnup, as the distribution of fissile material changes in the core's geometry over time which can cause unnecessary complications. Galahom et al. [8] at the Higher Technological Institute in Egypt tested the thorium fuel’s performance through Monte Carlo simulations at the Westinghouse Electric Company. Modeled in Figure 6, thorium fuels demonstrate a larger consumption of usable absorber pins, indicating that these localized burnups create environments of higher energy that are unsustainable for the absorber pins. The use of thorium presents challenges to optimize longevity cycles, plant safety and the need for additional design features.
While SMRs can employ a large range of fuel cells, each option faces unique challenges for optimization. Though current SMR models have solidified HALEU as a standard industrial fuel source, further research is required to defend the general dominance of radioactive fuels in modern energy production. Although fuel alternatives, like thorium and ceramic fuel cells, and their coupled technologies are still under development, continued exploration of these components is essential to improve the current energy crisis.
Hybrid Systems
SMRs are often considered as modular parts in systems with renewable energy grids. The versatility of thermal energy SMRs produce can address shortfalls where wind and solar resources are unpredictable. In 2023, Masotti et al. [9] at the Department of Energy in Italy studied this situation, where they simulated iPWR SMRs in hybrid energy systems.
Figure 7: The design of a NHES, in terms of both technologies and the capacities of interconnected subsystems [9].
The energy grid diagram in Figure 7 includes SMRs as an additional power source to produce hydrogen when electrical energy is insufficient. Simulations were run to evaluate energy output and costs. Although the simulated SMR output was unrealistically constant and lacked power variability or peaking capabilities, its thermal energy storage from PWR-based reactors balanced the changing outputs of wind and water resources and were able to meet production expectations. While the criteria for hydrogen production were met in the simulations, an economics analysis was not conducted in the profitability of the whole grid, demonstrating the potential role SMRs may take in current energy systems.
Conclusion
Ultimately, SMRs represent a significant step towards the pursuit of clean and sustainable energy. Their modularity, advanced safety systems, and innovative fuel sources make them a compelling and versatile tool in modern energy grids. From improving existing PWR designs and components, like absorber pins, to more drastic approaches such as the EM2s uncustomary cooling and fuel systems, the variety of SMR designs and options demonstrate the potential nuclear energy holds to accommodate a vast number of populations. Despite promising results, many of these technologies face challenges such as high initial costs, regulatory hurdles, and fuel optimization which remain significant roadblocks. Continued investments in private research and government-funded projects are crucial to bring out the full potential of SMRs, address global energy demands and replace the mainstream use of fossil fuel-based systems with greener technologies.
Dr. Raj Shah is a Director at Koehler Instrument Company in New York, where he has worked for the last 25 plus years. He is an elected Fellow by his peers at IChemE, ASTM,AOCS, CMI, STLE, AIC, NLGI, INSTMC, Institute of Physics, The Energy Institute and The Royal Society of Chemistry. An ASTM Eagle award recipient, Dr. Shah recently coedited the bestseller, “Fuels and Lubricants handbook”, details of which are available at ASTM’s Long-awaited Fuels and Lubricants Handbook https://bit.ly/3u2e6GY.
He earned his doctorate in Chemical Engineering from The Pennsylvania State University and is a Fellow from The Chartered Management Institute, London. Dr. Shah is also a Chartered Scientist with the Science Council, a Chartered Petroleum Engineer with the Energy Institute and a Chartered Engineer with the Engineering council, UK. Dr. Shah was recently granted the honorific of “Eminent engineer” with Tau beta Pi, the largest engineering society in the USA. He is on the Advisory board of directors at Farmingdale university (Mechanical Technology), Auburn Univ (Tribology), SUNY, Farmingdale, (Engineering Management) and State university of NY, Stony Brook (Chemical engineering/ Material Science and engineering). An Adjunct
Professor at the State University of New York, Stony Brook, in the Department of Material Science and Chemical Engineering, Raj also has over 680 publications and has been active in the energy industry for over 3 decades. More information on Raj can be found at https://shorturl.at/JDPZN
Contact: rshah@koehlerinstrument.com
Mr. Bradford Leung is part of a thriving internship program at Koehler Instrument company in Holtsville, and is a student of Chemical Engineering at Stony Brook University, Long Island, NY where Dr. Shah is the chair of the external advisory board of directors
Works Cited
[1] Liou, Joanne. “What Are Small Modular Reactors (SMRs)?” International Atomic Energy
Agency, 13 Sept. 2023, www.iaea.org/newscenter/news/what-are-small-modular-reactors-smrs.
[2] “Biden-Harris Administration Announces $900 Million to Build and Deploy Next-Generation Nuclear Technologies.” Energy.gov, 2024, www.energy.gov/articles/biden-harris-administration-announces-900-million-build-and-deploy-next-generation-nuclear.
[3] Allen, Myles, et al. “Summary for Policymakers.” Cambridge University Press, Cambridge University Press, 2022, www.cambridge.org/core/books/global-warming-of-15c/summary-for-policymakers/31C38E590392F74C7341928B681FF668.
[4] Vanatta, Max, et al. “Technoeconomic Analysis of Small Modular Reactors Decarbonizing Industrial Process Heat.” Joule, vol. 7, no. 4, 1 Apr. 2023, pp. 713–737, https://doi.org/10.1016/j.joule.2023.03.009. Accessed 3 May 2024.
[5] “Backgrounder on New Nuclear Plant Designs.” NRC Web, www.nrc.gov/reading-rm/doc-collections/fact-sheets/new-nuc-plant-des-bg.html.
[6] Bolukbasi, M.J., et al. “Shifting to a 36-Month Fuel Cycle with Advanced Moderating Burnable Absorbers Enabling High Assay Low Enriched Uranium (HALEU).” Progress in Nuclear Energy, vol. 168, Mar. 2024, p. 105011, https://doi.org/10.1016/j.pnucene.2023.105011. Accessed 17 Nov. 2024.[7] Amila Amatullah, et al. “Comparative Analysis on Small Modular Reactor (SMR) with Uranium and Thorium Fuel Cycle.” Nuclear Engineering and Design, vol. 418, 1 Mar. 2024, pp. 112934–112934, https://doi.org/10.1016/j.nucengdes.2024.112934. Accessed 19 Sept. 2024.
[8] Galahom, A. Abdelghafar, et al.‘Explore the Possible Advantages of Using Thorium-Based Fuel in a Pressurized Water Reactor (PWR) Part 1: Neutronic Analysis’. Nuclear Engineering and Technology, vol. 54, no. 1, 2022, pp. 1–10, https://doi.org/10.1016/j.net.2021.07.019.
[9] Masotti, Guido Carlo, et al.‘Modeling and Simulation of Nuclear Hybrid Energy Systems Architectures’. Energy Conversion and Management, vol. 298, 2023, p. 117684, https://doi.org/10.1016/j.enconman.2023.117684.
The content & opinions in this article are the author’s and do not necessarily represent the views of AltEnergyMag
Comments (0)
This post does not have any comments. Be the first to leave a comment below.
Featured Product
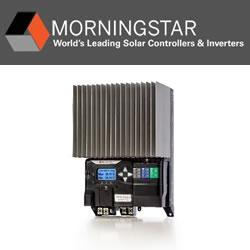