Recent advancements and developmental efforts have been made in nuclear energy, including advanced designs for nuclear plants/reactors, waste management, nuclear accidents, and more.
Recent Advancements in the Field of Nuclear Power
Dr. Raj Shah and Salowa Siddique | Kohler Instrument Company
Even though we are approaching a global energy crisis, reaching net zero emissions of greenhouse gases, decarbonizing electricity and heat production, and reducing the reliance on fossil fuels are also top energy security priorities. Among clean energy sources, nuclear energy has played an essential role in correcting environmental pollution and can be considered the backbone of producing low-carbon electricity generation. Among clean energy sources, nuclear power has the potential to produce vast amounts of energy. Along with electricity generation, nuclear energy has diverse applications in many industries. However, it comes with some disadvantages. Recent advancements and developmental efforts have been made in nuclear energy, including advanced designs for nuclear plants/reactors, waste management, nuclear accidents, and more.
Nuclear energy today and its importance
The exploration of peaceful applications of nuclear energy began after the devastating power of nuclear reactions was demonstrated by the development of the atomic bomb during World War 2.
Since then, nuclear energy has been vital in meeting the world’s growing energy demands. Some advantages of nuclear energy are low greenhouse gas emissions, reliable and stable energy supply, high energy density, energy security, and long-term sustainability. Even though solar PV is leading toward replacing fossil fuels, it needs to be complemented by dispatchable resources like nuclear power. Over the past 50 years, nuclear power has reduced CO2 emissions by over 60 Gigatons, which is nearly two years’ worth of global energy-related emissions. Nuclear energy contributes to energy security in multiple ways. Nuclear plants help to keep power grids stable. They can adjust their operations to follow demand and supply shifts to a certain extent. The need for such services will increase as the share of variable renewables like wind and solar photovoltaics (PV) rises. Nuclear plants can help to limit the impacts of seasonal fluctuations in output from renewables and bolster energy security by reducing dependence on imported fuels [1].
Consumption of nuclear energy
Nuclear energy is the world’s second-largest source of low-carbon power, providing about 10% of the world's electricity from about 440 power reactors. Today, the nuclear industry is characterized by international commerce. When the commercial nuclear sector began in the 1960s, there were clear boundaries between the industries of the East and West. A reactor under construction in Asia today may have components supplied from South Korea, Canada, Japan, France, Germany, Russia, and other countries. Similarly, uranium from Australia or Namibia may end up in a reactor in the UAE, converted in France, enriched in the Netherlands, deconverted in the UK, and fabricated in South Korea [2]. Countries that have kept the option of using nuclear power need to reform their policies to ensure competition on a level playing field. They must also address barriers to investment in lifetime extensions and new capacity. The focus should be on designing electricity markets to value low-carbon technologies' clean energy and energy security attributes, including nuclear power [3].
Figure 1: Nuclear electricity production by various countries from 1970-2021 [2].
Globally, efforts are being made to improve nuclear power development. Some examples of participating countries include Canada, Mexico, and the USA. Canada has 19 operable nuclear reactors, with a combined net capacity of 13.6 GWe. In 2021, nuclear generated 14.3% of the country's electricity. Mexico has two operable nuclear reactors with a collective net capacity of 1.6 GWe. In 2021, nuclear developed 5.3% of the country's electricity. The USA has 93 operable nuclear reactors, with a combined net capacity of 95.8 GWe. In 2021, nuclear generated 19.6% of the country's electricity [2]. Some recent data on developments in grid connections and the start of construction for reactors in different countries is provided below:
Figure 2: Developments in 2023 for grid connections and start of construction
As of May 2023, 436 nuclear reactors were in operation in 32 countries around the world, and the United States had the most significant number of nuclear power reactors in operation at the time, with 93 units. The largest reactors under construction as of May 2023 were situated in the UK, with a gross electricity generation capacity of 1,720 megawatts. China, having the largest number of nuclear reactors under construction worldwide, had a total capacity of almost 22 gigawatts [4]. Many countries have recently taken initiatives to extend operations at existing nuclear power plants and build new ones. This shows the world is slowly relying on nuclear power to produce reliable electricity on a large scale.
Advanced reactor designs
The construction of new and advanced nuclear power plants is increasing rapidly and aligning with the future net zero scenario. Some new nuclear technologies from different countries and regions include Belgium recently deciding to extend the operation of two existing reactors from 2025 to 2035, which will meet around 15% of electricity demand, Canada introducing an investment tax credit of up to 30% for clean energy technologies in late 2022, explicitly including small modular reactors (SMRs), Finland completing Olkiluoto 3 in 2023, the first new nuclear reactor in Western Europe in 15 years [1]. The U.S. Department of Energy's Advanced Reactor Demonstration Program (ARDP) is responsible for building advanced reactor designs that have diverse representation and the ability to have various applications. They range from advanced light-water-cooled small modular reactors to innovations that use molten salts and high-temperature gases to flexibly operate at even higher temperatures and lower pressures [5]. The innovative designs can compete globally and promise access to a reliable, clean power source and portable drinking water in disadvantaged communities. Three advanced reactor designs that have the potential to be operational in the upcoming years are:
BWXT Advanced Nuclear Reactor: It is a transportable microreactor that can be deployed in off-grid applications and remote areas, potentially producing 50 megawatts of thermal energy. Some future and ongoing research on this model includes testing and qualifying the fuel that will be used for high performance and optimizing new manufacturing technologies that would reduce the cost of microreactors and initiate or benefit other reactor designs.
Figure 3: Design of BWXT nuclear reactor [5].
Holtec SMR-160 reactor: This developing nuclear power plant consists of advanced light water small modular reactors providing thermal power of 160 Megawatts and can use air cooling condensers on the secondary side, making it suitable to be deployed in the most arid regions worldwide.
Figure 4: Design of Holtec SMR-160 nuclear power plant [5].
Molten Chloride Reactor Experiment: A small reactor experiment is being implemented with the molten chloride fast reactor (MCFR) technology. The MCFR can be scaled up for commercial use on the grid and operate on multiple fuels, including used nuclear fuel from other reactors. MCFR technology transfers heat incredibly efficiently and can be utilized for thermal storage, process heat, or electricity production. The molten chloride reactor experiment will inform a demonstration reactor's design, license, and operation and is expected to be operational within the next five years [5].
Figure 5: Design of molten chloride reactor technology.
Compared with previous nuclear reactors, the performance has improved substantially over time. Over the last 40 years, the proportion of reactors reaching high-capacity factors has increased significantly. For example, 68% of reactors achieved a capacity greater than 80% in 2021, compared to less than 30% in the 1970s. In contrast, only 6% of reactors had a capacity factor lower than 50% in 2021, compared to just over 20% in the 1970s [2].
Figure 6: Long-term trends in capacity factors
Waste management
The nuclear energy industry is crucial in meeting the world’s ever-growing energy demands. However, managing radioactive waste has been a multifaceted challenge that requires careful planning, rigorous regulations, and responsible practices. Effective and continuous management of nuclear waste is vital to ensure the continued use of nuclear energy as a low-carbon energy source and to move closer to the net zero scenario. Workable solutions have been made to deal with the issue. Typically, the amount of waste produced by the nuclear power industry is small relative to both other forms of electricity generation and general industrial activity. Nuclear power is also the only large-scale energy-producing technology that takes full responsibility for all its waste and fully costs this into the product.
The preferred alternative to reduce nuclear waste is to place it in sealed containers and store it underground. Some popular and recent methods of managing waste are:
Reprocessing used fuel: Reprocessing used fuel has been popular in many countries. Used nuclear fuel has been reprocessed to extract fissile materials for recycling and to reduce the volume of high-level waste. Several European countries, Russia, China, and Japan, have policies to reprocess used nuclear fuel [6]. Reprocessing allows for a significant amount of plutonium to be recovered from used fuel, mixed with depleted uranium oxide in a fabrication plant to make fresh fuel. This process allows some 25-30% more energy to be extracted from the original uranium ore, significantly reducing the volume of high-level waste by 85% [6].
Decommissioning nuclear plants: Although 99% of radioactivity is associated with fuel, the remaining radioactivity comes from activation products such as steel components, which have long been exposed to neutron irradiation. The atoms are changed into different isotopes such as iron-55, cobalt-60, nickel-63, and carbon-14, in which the first two are highly radioactive, emitting gamma rays. Some cesium-137 may also be found in decommissioning wastes. Scrap material from decommissioning may be recycled, but for uses outside the industry, very low clearance levels are applied, so most are buried, and some are recycled within the industry [6].
Storage ponds: Deep geological disposal is the preferred option in most countries. At present, there are about 263,000 tonnes of used fuel in storage. Over two-thirds of this is in storage ponds, with an increasing proportion in dry storage. A storage pond, CLAB in Sweden, is 7-12 meters deep and allows several meters of water to run over the used fuel. The multiple racks are made of metal with neutron absorbers incorporated. The circulating water both shields and cools the fuel. These pools are robust constructions made of thick reinforced concrete with steel liners. Ponds at reactors are often designed to hold all the used fuel produced over the planned operating lifetime of the reactor [6].
Figure 7: Storage pond of Central Interim Storage Facility for Spent Nuclear Fuel (CLAB) facility in Sweden [6].
International Collaboration and further research
In addition to commercial nuclear power plants, about 220 research reactors operate in over 50 countries, with more under construction. As well as being used for research and training, many reactors produce medical and industrial isotopes. The use of reactors for marine propulsion is mostly confined to the major navies, where it has played an important role for five decades, providing power for submarines and large surface vessels [2]. The widespread use of nuclear power started being implemented long ago. The largest numbers of reactors as of 2010 were in the United States (104), France (58), and Japan (54).
Figure 8: Nuclear power plants under construction in 2010 [8].
As of 2023, more participating countries are willing to express interest in introducing nuclear power. Notably, in the 1980s, 218 power reactors started up, an average of one every 17 days. These included 47 in the USA, 42 in France, and 18 in Japan. The average power was 923.5 MWe. So, it is easy to imagine a similar number being commissioned in a decade after about 2015 [8].
Figure 9: Net electrical capacity and number of reactors under construction as of 2023 [9].
Some other initiatives that are being taken to make advanced nuclear projects a reality are working on fourth-generation reactor designs that will vary in size, offering enhanced versatility and being more affordable to build and operate. The Advanced Research Projects Agency-Energy (ARPA-E) is creating new power plants on time and developing new technologies to help lower the cost of building advanced reactor systems. ARPA-E also aims to develop breakthrough technologies that will help facilitate a ten-times reduction in the volume of spent nuclear fuel required for permanent disposal. Many project teams are focused on improvements to fuel recycling, safeguards in accounting for nuclear materials, and developing high-performance waste forms across multiple reactor classes [9].
Conclusion
Nuclear power provides about 10% of the world's electricity and 18% of electricity in OECD countries. Almost all reports on future energy supply from major organizations suggest an increasing role for nuclear power as an environmentally benign way of producing reliable electricity on a large scale. The future of nuclear energy in a carbon-constrained world promises a big portion of low-carbon electricity. Energy consumption in the form of electricity is expected to increase from about 20% today to about 50% by 2050. While absolute supply from nuclear increases, its relative contribution to the electricity mix is projected to decrease from about 10.5% in 2020 to about 8% in 2050 [8]. With the new generation of new reactor designs and new waste management strategies, transitioning into clean energy is reachable in upcoming years.
About the Authors
Dr. Raj Shah is a Director at Koehler Instrument Company in New York, where he has worked for the last 28 years. He is an elected Fellow by his peers at IChemE, CMI, STLE, AIC, NLGI, INSTMC, Institute of Physics, The Energy Institute and The Royal Society of Chemistry. An ASTM Eagle award recipient, Dr. Shah recently coedited the bestseller, “Fuels and Lubricants handbook”, details of which are available at ASTM’s Long-Awaited Fuels and Lubricants Handbook 2nd Edition Now Available (https://bit.ly/3u2e6GY).He earned his doctorate in Chemical Engineering from The Pennsylvania State University and is a Fellow from The Chartered Management Institute, London. Dr. Shah is also a Chartered Scientist with the Science Council, a Chartered Petroleum Engineer with the Energy Institute and a Chartered Engineer with the Engineering council, UK. Dr. Shah was recently granted the honorific of “Eminent engineer” with Tau beta Pi, the largest engineering society in the USA. He is on the Advisory board of directors at Farmingdale university (Mechanical Technology) , Auburn Univ ( Tribology ), SUNY, Farmingdale, (Engineering Management) and State university of NY, Stony Brook ( Chemical engineering/ Material Science and engineering). An Adjunct Professor at the State University of New York, Stony Brook, in the Department of Material Science and Chemical engineering, Raj also has over 585 publications and has been active in the alternative energy industry for over 3 decades.
Ms. Salowa Siddique is a part of a thriving internship program at Koehler Instrument company in Holtsville, and are students of Chemical Engineering at Stony Brook University, Long island, where Dr. Raj Shah is the chair of the External Advisory Board of Directors.
References
- IEA (2019), Nuclear Power in a Clean Energy System, IEA, Paris https://www.iea.org/reports/nuclear-power-in-a-clean-energy-system, License: CC BY 4.0
- Nuclear Power Today | Nuclear Energy - World Nuclear Association, world-nuclear.org/information-library/current-and-future-generation/nuclear-power-in-the-world-today.aspx#:~:text=Nuclear%20energy%20now%20provides%20about,in%20about%20220%20research%20reactors. Accessed 11 Sept. 2023.
- IEA (2019), Nuclear Power in a Clean Energy System, IEA, Paris https://www.iea.org/reports/nuclear-power-in-a-clean-energy-system, License: CC BY 4.0
- Published by Statista Research Department, and Aug 29. “Nuclear Power Plants in the World 2023.” Statista, 29 Aug. 2023, www.statista.com/statistics/267158/number-of-nuclear-reactors-in-operation-by-country/.
- Advanced Reactor Designs to Watch in 2030. Energy.Gov, www.energy.gov/ne/articles/5-advanced-reactor-designs-watch-2030. Accessed 11 Sept. 2023.
- Radioactive Waste Management | Nuclear Waste Disposal - World Nuclear Association, world-nuclear.org/information-library/nuclear-fuel-cycle/nuclear-wastes/radioactive-waste-management.aspx. Accessed 11 Sept. 2023.
- Ahearne, J. F. (2011). Prospects for nuclear energy. Energy Economics, 33(4), 572-580. https://doi.org/10.1016/j.eneco.2010.11.014
- World Energy Needs and Nuclear Power | Energy Needs | Nuclear Energy Meeting Energy Needs - World Nuclear Association, world-nuclear.org/information-library/current-and-future-generation/world-energy-needs-and-nuclear-power.aspx. Accessed 11 Sept. 2023.
- 3 Early-Stage R&D Programs Transforming the Nuclear Industry. Energy.Gov, www.energy.gov/ne/articles/3-early-stage-rd-programs-transforming-nuclear-industry. Accessed 11 Sept. 2023.
The content & opinions in this article are the author’s and do not necessarily represent the views of AltEnergyMag
Comments (0)
This post does not have any comments. Be the first to leave a comment below.
Featured Product
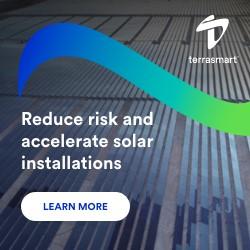